Smart fabrics and self-powered sensing
Smart fabrics and wearable electronics can be developed using highly conductive and stretchy fibers. Most of these fiber conductors are, however, strain sensitive with limited conductance on stretching. As a result, a new strategy can be introduced by rearranging the geometry of the conductive path for stable conductance. In a new report now published on Science Advances, Lijing Zheng and colleagues in China and Germany, described a coaxial wet-spinning process to continuously develop intrinsically stretchable and highly conductive, yet conductance-stable liquid metal (LM) sheath-core microfibers. The team stretched the microfibres up to 1170 percent and fully activated the conductive path to obtain a very high conductivity of 4.35 x 104 S/m and a resistance change of only 4 percent at 200 percent strain. The microfiber could be woven easily into everyday glove fabrics and as excellent joule heaters, electro-thermochromic displays and self-powered wearable sensors.
Stretchable fiber conductors
Stretchable fiber conductors can be easily developed into fabrics with high air permeability and can be well integrated as wearable sensors with increasing interest. Stretchable conductive fibers have highly sensitive conductance changes and promote stable conductance. Recent developments in high-performance electronics have a high demand for stretchable fiber electrodes or interconnects to steadily transport electrical signals between active electronic components without notable conductance loss. To overcome existing limits, Zheng et al. embedded deformable conductive fillers with strain-enhanced conductivity into an elastic matrix to produce ultralong, intrinsically stretchable fiber conductors with stable and high conductance. The research team proposed a coaxial wet-spacing method to prepare super-elastic liquid metal sheath-core microfibers with high and ultra-stable conductance. They then explored the promising applications of the liquid metal sheath-core microfibers in smart fabrics and self-powered sensing processes, relative to joule heating, electrothermochromism and triboelectric properties.
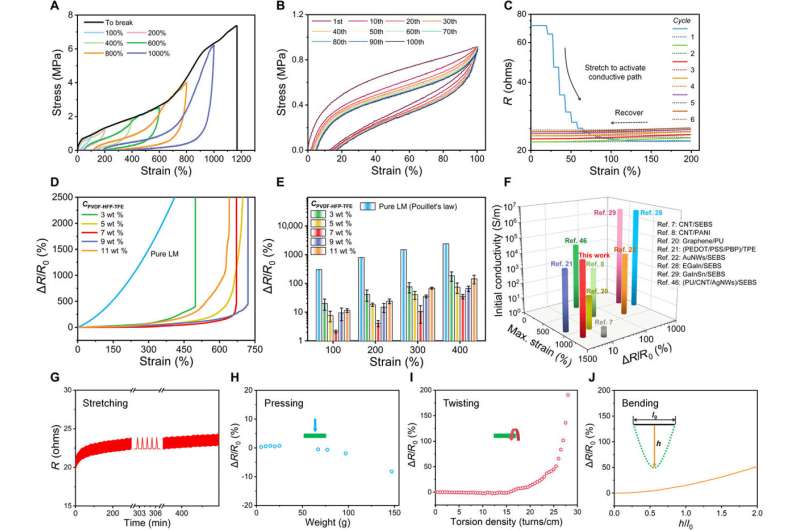
The experiments—preparing the liquid-metal (LM) sheath-core microfibers.
Zheng et al. used coaxial wet-spinning to prepare liquid metal sheath-core microfibres and improved the fiber quality by smoothly solidifying the spinning solution. They used three spinning solutions in the inner channel and distilled water in the outer channel as the coagulating bath. The team also introduced a covalent network in the sheath post-ultraviolet polymerization to improve the toughness and elastic recovery of the LM sheath-core fiber. Based on the strategy, Zheng et al. could continuously wet-spin the LM sheath-core microfibers into an ideally unlimited length. After fully drying the microfiber, they imaged the product using scanning electron microscopy (SEM) to observe a uniform and regular surface. The team next sowed good electrical conductivity of the fibers using a light-emitting diode (LED).
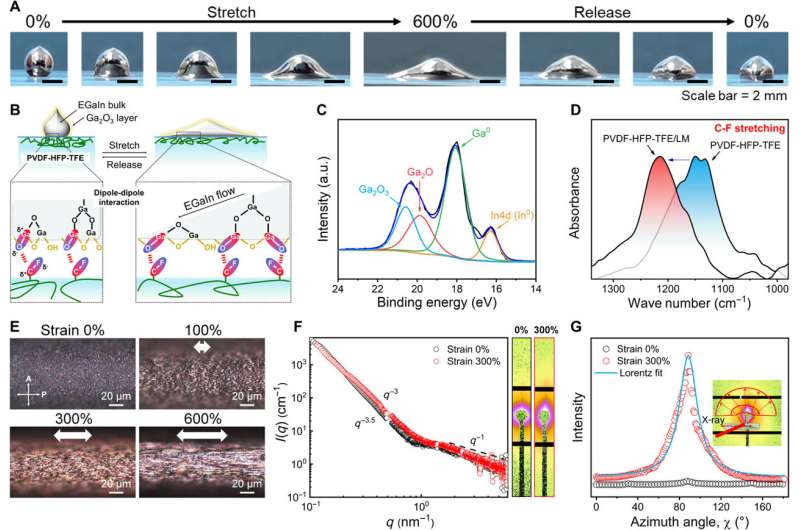
Mechanical properties and strain-insensitive conductance
To understand the highly elastic stretchability and cyclability of LM sheath-core microfibers, the scientists next conducted cycling tensile tests to note good elastic recovery of 100 percent after 100 cycles. To impart electric conductivity on the LM sheath-core microfiber, Zheng et al. next used a sequential freezing-plus-stretching strategy for conductivity activation. The resulting excellent strain-insensitive conductance of the microfiber contributed to further studies on the stability and durability of electrical properties in real-time, for practical applications within smart fabrics and wearable electronics. To fully sinter the liquid-metal particles for activated conductivity, the scientists used a sequential ‘freezing-plus-stretching’ strategy. Due to its abnormal volume expansion behavior, the LM expanded instead of shrinking at low temperatures. As a result, the liquid nitrogen freezing-induced phase and stiffness change assisted the LM particles to pierce through the oxide and elastomer coating for partial activation of the conductive path. Subsequent mechanical stretching of the material to 200 percent reduced the resistance to allow a considerably high conductivity. Repeated stretching and recovery showed a small amplitude of resistance changes for the successful construction of stable and continuous conductive networks. Based on the excellent strain-insensitive conductance of the microfiber, Zheng et al. studied the stability and durability of the electrical properties in real-time applications. The results highlighted the high and strain-insensitive conductance of LM sheath-core microfibers that were not largely affected by deformation, with practical applications in smart fabrics and wearable electronics.
Mechanisms of strain-insensitive conductance
To understand the mechanism of excellent conductance within the LM sheath-core microfiber, Zheng et al. next investigated the interaction between the fluoroelastomer and the liquid-metal boundary. The liquid metal maintained very high surface tension and underwent fully recoverable shape deformation to suggest that LM could reconciliate its surface to form a firm interface with the fluoroelastomer during strain-insensitive conductance. The team supported the presence of the surface oxidation layer on the LM particles using a 3D X-ray photoelectron spectroscopy (XPS) spectrum. The results showed the important role of the dipolar attraction between the LM oxidation layer and fluoroelastomer during LM deformation and high conductance tolerance. The team then used the small-angle X-ray scattering method to further investigate the small microstructural changes of the fiber.
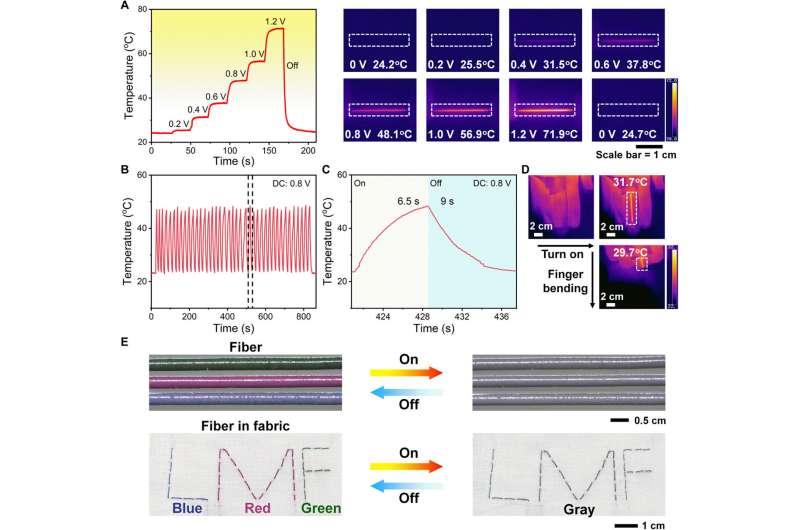
Joule heating effect
The scientists next studied the potential applications of integrating the LM sheath core microfibers in smart fabrics. Due to the fine nature of the fiber with a small Young’s modulus, it wasn’t feasible to integrate the fiber with fabrics, since it could not influence the mechanical properties of the fabric. The fiber could, however, be used as an electrical heater via the joule heating effect due to its strain-insensitive conductance. For instance, the temperature of the fiber increased uniformly and simultaneously with an applied DC voltage. The team also embedded the LM sheath-core microfiber into an elastic spandex glove and demonstrated multiple findings with smart fabrics to prove the microfiber’s potential during intelligent display and adaptive camouflage. The LM sheath-core microfibers also acted as ideal, highly stretchable self-powered sensors based on the single-electrode-mode triboelectric mechanism. The ultrasmall Young’s modulus and ultrahigh stretchability of the LM sheath-core microfibers made them suitable for imperceptible wearable sensing. The team repeatedly contacted the LM sheath-core microfiber with diverse materials including cotton, silk and aluminum foil to monitor the induced voltage and current. During skin contact on the human wrist, the fiber demonstrated powerful sensing potential as a self-powered wearable sensor.
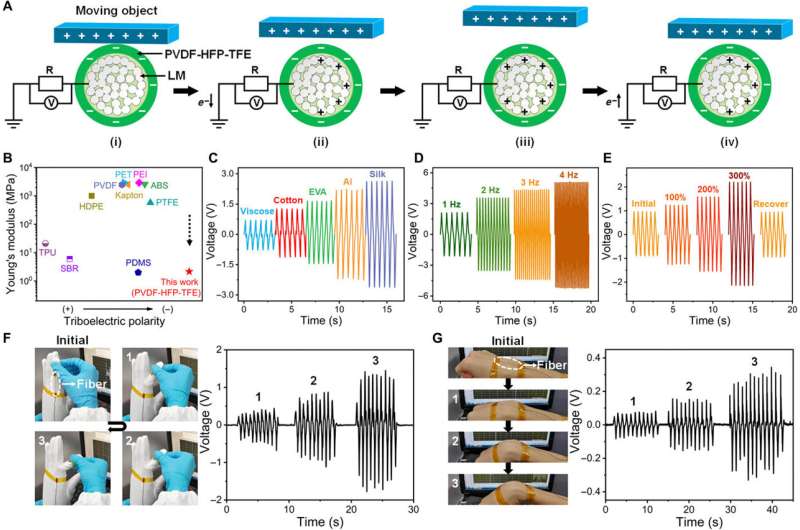
Outlook
In this way, Lijing Zheng and colleagues used coaxial wet-spinning based on liquid metal particles and polymer materials to develop a highly conductive, intrinsically stretchable LM sheath-core microfibers with high stretchability and strain-insensitive conductance. The team noted applications of the microfibers during joule heating, electrothermochromism and self-powered sensing for potential applications with smart textiles and wearable sensors.
1. Zheng L. et al. Conductance-stable liquid metal sheath-core microfibers for stretchy smart fabrics and self-powered sensing, Science Advances, DOI: 10.1126/sciadv.abg4041
2. Tan J. Y. et al. A transparent, self-healing and high-κ dielectric for low-field-emission stretchable optoelectronics, Nature Materials, 10.1038/s41563-019-0548-4
3. Liu Z. F. et al. Hierarchically buckled sheath-core fibers for superelastic electronics, sensors, and muscles, Science, 10.1126/science.aaa7952
© 2021 Science X Network
Citation:
Smart fabrics and self-powered sensing (2021, June 15)
retrieved 15 June 2021
from https://techxplore.com/news/2021-06-smart-fabrics-self-powered.html
This document is subject to copyright. Apart from any fair dealing for the purpose of private study or research, no
part may be reproduced without the written permission. The content is provided for information purposes only.
For all the latest Technology News Click Here
For the latest news and updates, follow us on Google News.